New coronavirus variants are emerging across the globe: Everything we know
Scientists are just beginning to understand how mutations to the coronavirus may affect spread, immunity and vaccines.
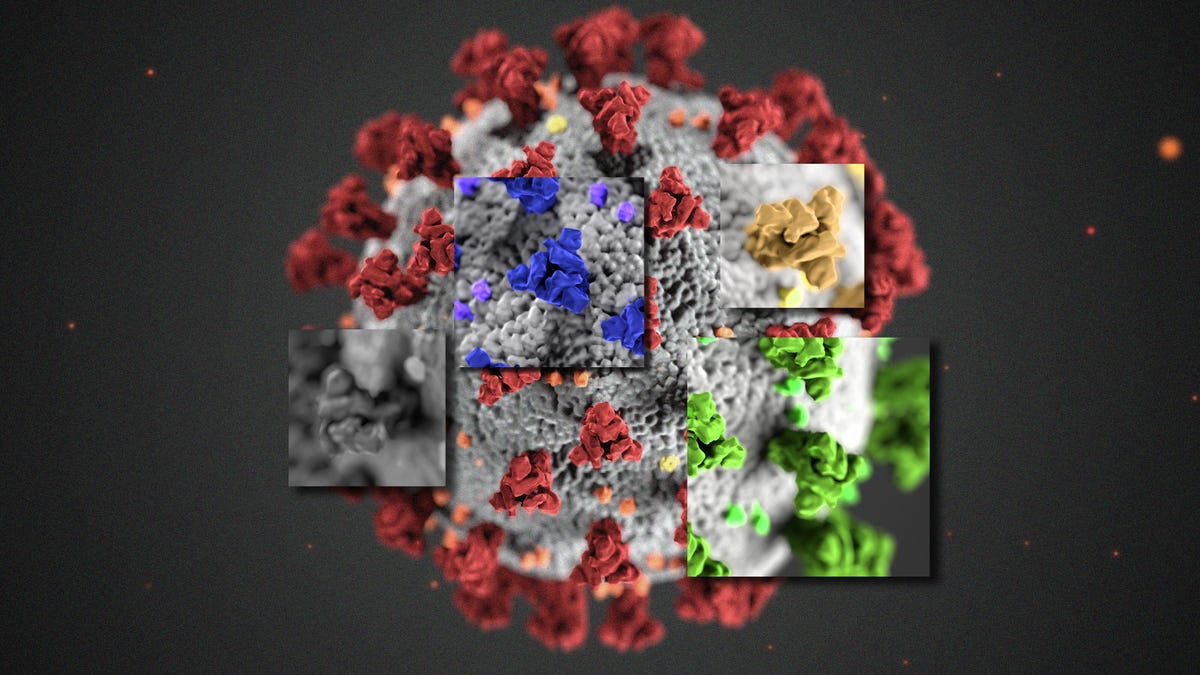
The coronavirus SARS-CoV-2 has constantly evolved since it was first detected in humans over a year ago. Viruses replicate exceedingly fast, and each time they do, there's a small chance they mutate. This is par for the course, if you're a virus .
But in the last few weeks, scientists have been investigating SARS-CoV-2 variants with a handful of mutations arising much faster than expected. Normally, we'd expect to see one to two largely inconsequential genetic changes in the coronavirus every few months. New variants are emerging with a constellation of mutations, all at the same time.
In December 2020, the UK announced a variant of coronavirus, and two other variants were later detected in South Africa and Brazil. There is, for the time being, no reason to fear these variants or how the coronavirus is mutating -- scientists and the World Health Organization suggest that our current protective measures of social distancing and masking up work just as well against them. However, scientists are closely monitoring and evaluating them because they could worsen the pandemic if they are more transmissible or can evade our immune system and vaccines.
Epidemiologists, virologists and immunologists are now tasked with understanding how these mutations in the new variants may change the virus and how our bodies respond to them. Mutations could change SARS-CoV-2 in such a way that it may even be able to evade the immune response generated by vaccines. Preliminary research shows current vaccines should be able to deal with the three most concerning variants, but data continues to roll in. On Sunday, South Africa temporarily halted the use of the AstraZeneca-Oxford vaccine after a small clinical trial found it didn't protect shot recipients from a fast-moving variant of the virus first discovered in the country.
Scientists can see the virus evolving in real time and are in a race to describe how this evolution might affect our immunity and, down the line, treatments and vaccines. Here, we're sharing everything we know about COVID-19 variants and the various esoteric ways scientists discuss mutations and evolution.
How does the coronavirus mutate?
The coronavirus is an RNA virus, which means its complete genetic sequence, or genome, is a single-stranded template (humans and other mammals, by contrast, use double-stranded DNA). The template of SARS-CoV-2 is made up of four bases -- denoted by the letters a, c, u and g -- in a specific sequence, about 30,000 letters long.
The template provides instructions on how to build all the proteins that make a new coronavirus particle. To replicate, SARS-CoV-2 needs to take over a host cell and use it as a factory, hijacking the machinery within. Once it sneaks into a cell, it needs to read the RNA template.
Critical to this process is an enzyme known as an RNA-dependent RNA polymerase, or RdRp. It has one job, and it's terrible at it. "This is an enzyme that makes a huge amount of mistakes when replicating," says Roger Frutos, a molecular microbiologist at the French Agricultural Research Centre for International Development, or CIRAD. The RdRp introduces errors during replication, producing new viruses with slightly different templates. Changes in the template are known as mutations.
Mutations often have little effect on a virus, but sometimes they change the template so much they cause changes in the virus' physical structure. "A mutant doesn't mean it's like 10 times scarier or 10 times deadlier," says Tyler Starr, a computational biologist at the Fred Hutchinson Cancer Research Center. "Mutations have incremental effects."
This could be a bad thing for SARS-CoV-2, creating a useless zombie virus. Sometimes, it might confer an advantage, like allowing the virus to bind more tightly to a host cell or helping it evade the immune response.
Scientists and researchers spot mutations by sequencing SARS-CoV-2 isolated from patients, looking at the entire 30,000 letters of its genome. They compare this with the earliest viruses on record, those detected in Wuhan, China, patients back in December 2019, and see how they've changed. "We never see viruses now that look exactly like what was in Wuhan," says Stuart Turville, an immunovirologist at the Kirby Institute in Australia.
If researchers see that a mutation is becoming more prevalent in a population, there's a chance it may have changed the characteristics of SARS-CoV-2.
What are the coronavirus variants?
Any mutations to the coronavirus genome results in variants of the virus, but some are more concerning than others. In late 2020, three variants were identified with mutations that may make SARS-CoV-2 more transmissible or, in the case of one variant, more deadly.
The variants are described by a number of names, which makes things a little confusing, but scientists refer to them by their lineage, giving them a letter-based descriptor based on their ancestry. They are:
- B.1.1.7, which was first detected in England in September 2020 and which has been detected in dozens of nations, including the US. A new study reinforces the prediction that this variant will dominate COVID-19 cases by March.
- B.1.351, first detected in South Africa and now found in more than 20 countries. It was detected in the US on Jan. 28.
- P.1, detected in Manaus, in the Brazilian state of Amazonas, and also discovered in Italy, South Korea and the US.
These will not be the last variants of SARS-CoV-2 that arise, and scientists continue to track changes in the genome. Any changes can be useful for genomic epidemiologists to assess transmission dynamics and patterns, in turn helping inform public health units to alter their response to any emerging threats. "We are watching all the time," says Catherine Bennett, chair in epidemiology at Deakin University in Australia.
But why are these three variants of particular concern? They share common characteristics that early analysis suggests may enable them to spread more easily or evade the immune response. This seems to result from, at least partially, how these mutants change the structure of the SARS-CoV-2 spike protein, which enables the virus to hijack cells and turn them into factories.
Could coronavirus variants change the efficacy of our vaccines? Scientists are trying to figure that out.
How do mutations cause structural changes?
Each SARS-CoV-2 particle is covered with spikes. Infiltration of a cell requires the club-like projections to lock onto a protein on the surface of a human cell known as ACE2, which facilitates viral entry.
But the viral protrusions are also recognized by the human immune system. When immune cells detect the SARS-CoV-2 spike, they begin pumping out antibodies to prevent it from locking on to ACE2, or send other cells in to destroy the virus. Antibodies also attach to the spike and can effectively prevent it from attaching to a cell. This puts the spike under extreme evolutionary pressure. Mutations that change the spike and help it evade immune cells or antibodies or lock onto ACE2 more strongly can provide a survival advantage.
The variants listed above all share mutations in a region of the spike known as the receptor binding domain, which directly contacts ACE2. If mutations cause structural changes in the RBD, it might bind to ACE2 differently and could, for example, prevent the immune system from recognizing it as dangerous.
Interlude: Amino acids
Here's where things get a little confusing, but it's important to understand how scientists denote specific mutations and why you're seeing all these numbers and letters flying around.
Remember that each RNA genome (the template) contains four molecular bases denoted by the letters a, c, u and g. When this template is read, every three-letter combination or "codon" (GAU, for instance) corresponds to an amino acid. A chain of amino acids becomes a protein.
But here's the confusing bit: Amino acids are also denoted by a single-letter code, unrelated to the RNA template letters. The amino acid alanine, for instance, is A. Aspartic acid is D. Glycine is G.
Why is this important? Because scientists discuss and study coronavirus mutations at the amino acid level.
For example, we've already seen one SARS-CoV-2 variant arise and come to dominate across the world.
Sometime in early 2020, the coronavirus picked up a mutation that resulted in an increase in infectivity. A mutation in the RNA template flipped an "a" to a "g," which caused a different amino acid to form in the RBD of the spike. This change was beneficial for the virus, and now it's the dominant form we see across the world.
The mutation is known as D614G. This notation, letter-number-letter, corresponds to a change in the amino acid at position 614, from aspartic acid (D) to glycine (G).
Confusing? Definitely. Important? Absolutely. This naming convention is important to understand important mutations in the three new COVID-19 variants.
Strengthening lockdowns in the UK has helped curb the spread of the variant, B.1.1.7
Which coronavirus mutations concern scientists most?
There are a number of mutations in all three variants across the RNA genome, but let's focus on the spike here. B.1.1.7 has eight mutations in its spike, B.1.351 has seven and P.1 has 10. Not all of these mutations are the same, but some overlap -- that is, the virus has evolved similar mutations in different locations across the world, a process known as "convergent evolution."
There are three mutations, all found in the RBD of the spike, which may affect the virus or how our antibodies respond to an infection:
- N501Y
- E484K
- K417N/T
Scientists are only just beginning to understand how these individual changes may benefit SARS-CoV-2 and if they're increasing its infectivity and transmissibility or making them more prone to evading the immune response. There's emerging evidence that, alone, they may not be significant changes -- but when found in combination with other mutations, they may facilitate more worrying differences from "original" SARS-CoV-2.
N501Y is found in all variants and is one of the mutations scientists are most interested in.
The change from an asparagine (N) to a tyrosine (Y) has been shown to increase SARS-CoV-2's ability to bind to ACE2 and, in mice, increase its infectivity. It's currently unknown whether this one change would elicit any changes in the mortality or morbidity of COVID-19. However, the change does not seem to impact the ability for the Pfizer/BioNTech vaccine to stimulate antibodies, according to preliminary research published on preprint server bioRxiv. That's good news.
In addition to N501Y, the B.1.351 and the P.1 variants have two more mutations: E484K and K417N/T, both of which change how sensitive the virus is to antibodies. These changes are slightly more concerning.
The two mutations are in regions of the RBD that antibodies can bind to. Researchers are concerned about E484K in particular and mutations at this site can reduce the neutralizing ability of antibodies more than 10 times. This could have the greatest impact on generating immunity, according to a preprint paper published on Jan. 4. Another preprint, published on Jan. 26, points to E484K as a key mutation in diminishing antibody activity against COVID-19. Worryingly, the mutation appears in 100% of cases infected with the P.1 variant -- and scientists are concerned it's allowing for a significant number of reinfections in Brazil.
The amino acid change at 417 is also interesting. In the South African B.1.351 variant, it's K417N. In the P.1 variant it's K417T. The amino acid change is different, but it appears to result in a similar effect -- improving evasion from antibodies. Preliminary studies reveal that position K417 is an important target of neutralizing antibodies, too, suggesting that both mutations could help the virus evade vaccine-mediated and naturally acquired immunity.
The UK government has also seen the E484K mutation in at least 43 cases, according to a recent technical briefing and the BBC.
Surveilling variants
These are merely three of the many mutations scientists are finding in the new variants -- how they all fit together in reality is much more complicated, and many more mutations that change SARS-CoV-2 are waiting to be discovered. For instance, a paper published on Jan. 28 in Cell discusses the N439K variant and its ability to evade antibodies.
Fortunately, scientists can get ahead of these variants by studying mutations that may occur in SARS-CoV-2. This is central to work performed by Starr and some of his colleagues at the Fred Hutchinson Cancer Research Center. "We've been generating these maps where we just survey all the possible mutations that could occur in the RBD," Starr says.
When a new variant arises, other researchers can look to these maps and see how the mutation affects the biochemical properties of the virus. Does it bind better? Worse? Is it more likely to evade the immune system? Starr explains this work has allowed for mapping how mutations might avoid treatments, like those used by Regeneron or Eli Lilly and can inform surveillance and response to emerging variants.
Maps like these, produced by the Bloom lab at the Fred Hutchinson Cancer Research Center, guide research on mutations. At significant sites in the RBD, the team analyzes how mutants change the binding affinity. Blue is increased affinity, red is decreased. The N501Y mutant is a deep blue, showing how this mutant has increased binding affinity to ACE2.
Should you be worried about coronavirus variants?
Presently, there's not enough evidence to suggest the variants are causing more significant mortality or more severe disease -- which means public health advice is largely unchanged. Wearing masks, social distancing and good hand and respiratory hygiene are the best way to prevent the spread of the disease. The coronavirus has not mutated to overcome these measures.
A more pressing question is how the variants and their mutations could affect vaccines and treatments and whether they'll increase the rate of reinfection. Vaccines stimulate immunity by showing the body a harmless version of the virus, which can produce antibodies that roam our inner halls looking for invaders. These antibodies may not be adept at catching and neutralizing variants, as explained above -- but researchers don't have a great handle on the data at present.
Even so, vaccine manufacturers have begun to plan for variants that negatively affect the immune response. A report in Science on Jan. 26 highlights Moderna's efforts to look ahead and potentially change the formulation of their mRNA vaccine and provide "booster" shots that could protect against new variants that may arise.
On Jan. 28, biotech firm Novavax released news of results from late-stage clinical trials of its own vaccine candidate. The trial was conducted on patients in both the UK and South Africa, with mixed results. In the UK, Novavax claims its vaccine had around 89.3% efficacy, but in South Africa, where the more evasive variant is circulating, this efficacy dropped to 60%. This result is concerning and makes an urgent case to evaluate our current vaccines against the newly emerged variants.
Additionally, if the variants infect someone who has previously been infected by COVID-19, there's a chance the immune system will not mount an adequate response and block infection. There's limited data on this, though the P.1 variant has been detected in a case of reinfection in Brazil. The patient was exposed to an earlier variant of SARS-CoV-2, but then acquired P.1 and scientists believe they may have gone through a second period where they were able to transmit the disease again. More work is required to fully understand this phenomenon.
Ultimately, COVID-19 continues to spread across the globe and more new infections means more opportunities for SARS-CoV-2 to evolve. The virus can't evolve without us -- indeed, it can't survive without us. The simplest way to prevent new variants from emerging is preventing the virus from spreading at all. Our efforts will need to be focused on speeding up the vaccine rollout across the globe and continuing to practice the distancing and hygiene measures we're already adept at.